Eric Mittelstaedt & Aurore Sibrant
University of Idaho
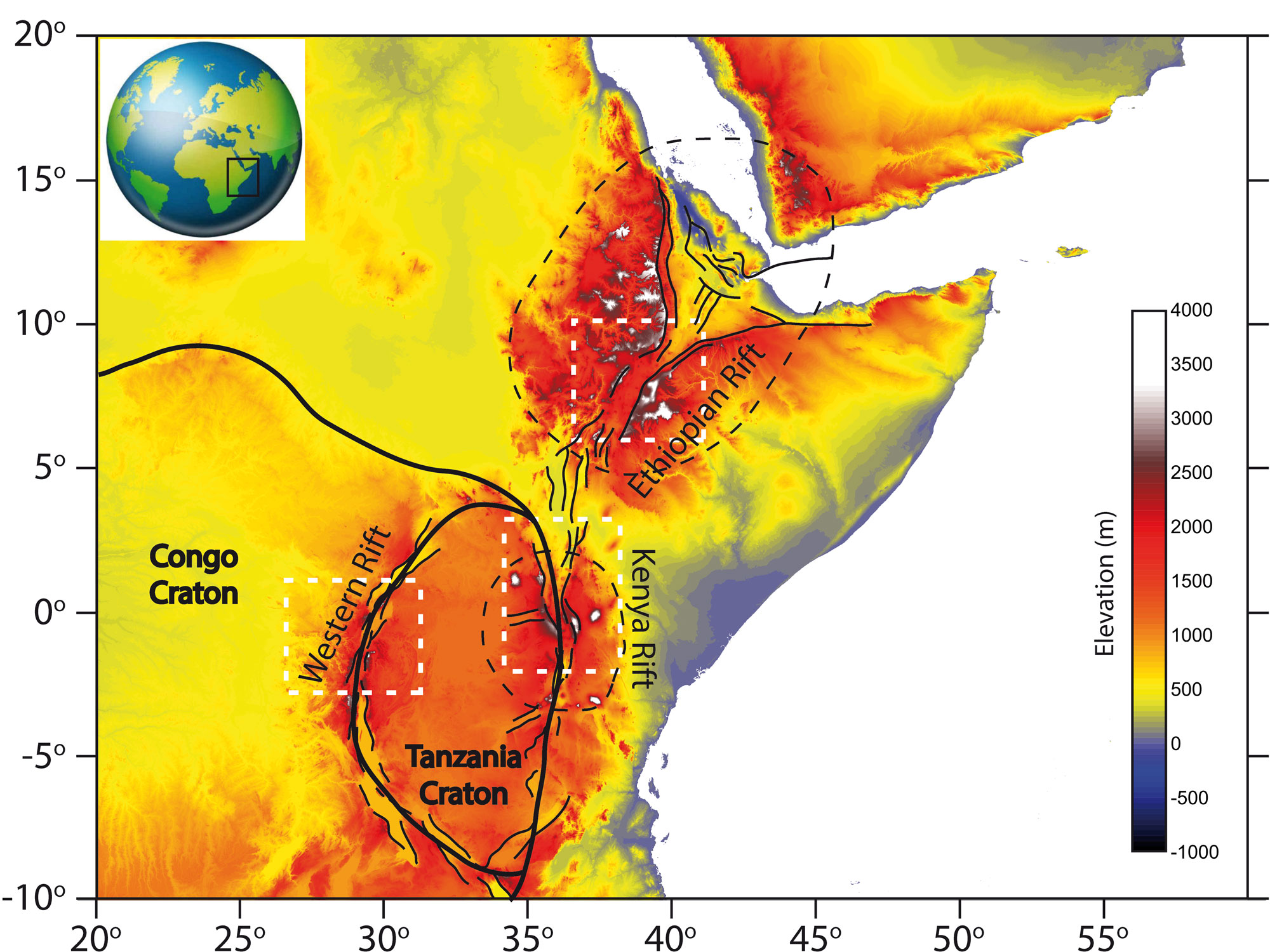
Figure 1. Digital Elevation model of the eastern part of Africa showing the main part of the East African Rift System (EARS). Solid black lines show major faults bounding rift depressions. The white dashed square shows the location of the focus rift and the black dashed elliptical lines indicates Ethiopian and Kenya domes. The thick black lines indicate the boundaries of the Congo and Tanzanian Craton.
The spatial variation in magma supply within a continental rift may determine the mode of lithospheric extension (active or passive) and the eventual pattern of oceanic spreading center segmentation (e.g., Hammond et al., 2013). As continental rifts evolve, volcanic centers within rift valleys often develop a characteristic spacing, or wavelength, such as observed in the Red Sea Rift (e.g., Bonatti, 1985) and within the Afar depression, the Main Ethiopian Rift (MER), and the Kenya (Gregory) Rift of the East African Rift System (EARS) (Fig. 1, 2; e.g., Mohr and Wood, 1976). Based primarily on observations, the surprisingly regular spacing of the volcanic centers within the EARS has been attributed to lithosphere thickness (Vogt, 1974; Mohr and Wood, 1976), pre-existing fault systems, and mantle processes similar to those at island arc and mid ocean ridges (Keer and Lister, 1988). In this project, we investigate the processes that control the spacing of volcanoes in the EARS. We are using numerical experiments to investigate if the surface expression of volcanism is primarily controlled by melt production (e.g., localized mantle instability, variations in mantle temperature and/or buoyancy) or by melt extraction (e.g., thickness of the lithosphere, pre-existing fractures).
The EARS is a perfect natural laboratory to test relationships between volcanism and parameters such as mantle temperature, lithosphere thickness, rift extension rate, and the presence of pre-existing structures. For example, the presence of one or two mantle plumes (Ebinger and Sleep, 1998; George et al., 1998) located primarily under the eastern rather than the western branch (e.g., Mulibo and Nyblade, 2013) suggests a role for anomalously warm, perhaps volatile-rich, mantle controlling the development of volcanic structures beneath the eastern branch rift segments. Additionally, decompression melting of upwelling mantle should be greater beneath the MER, where the opening rate is ~5 mm/yr (Saria et al., 2014), than beneath the Kenya rift, where the opening rate is ~3 mm/yr (Jestin et al., 1994; Saria et al., 2014). Differences in such tectonic and mantle parameters likely regulate magma supply throughout the EARS.
To constrain our experiments, we first examined the distribution of volcanoes throughout the EARS. We find that the median spacing of volcanoes in the Ethiopian and Kenya Rifts are similar (25 km and 32 km, respectively) and relatively uniform (e.g., small inter-quartile ranges, 15-16 km; Fig. 2). The median spacing of volcanoes in the Western Rift is much larger (53 km) and more irregular with an inter-quartile range of 68 km. We also found that volcano spacing may have some correlation with edifice volume, which could indicate a contribution of lithosphere flexure (e.g., Hieronymus and Bercovici, 1999).
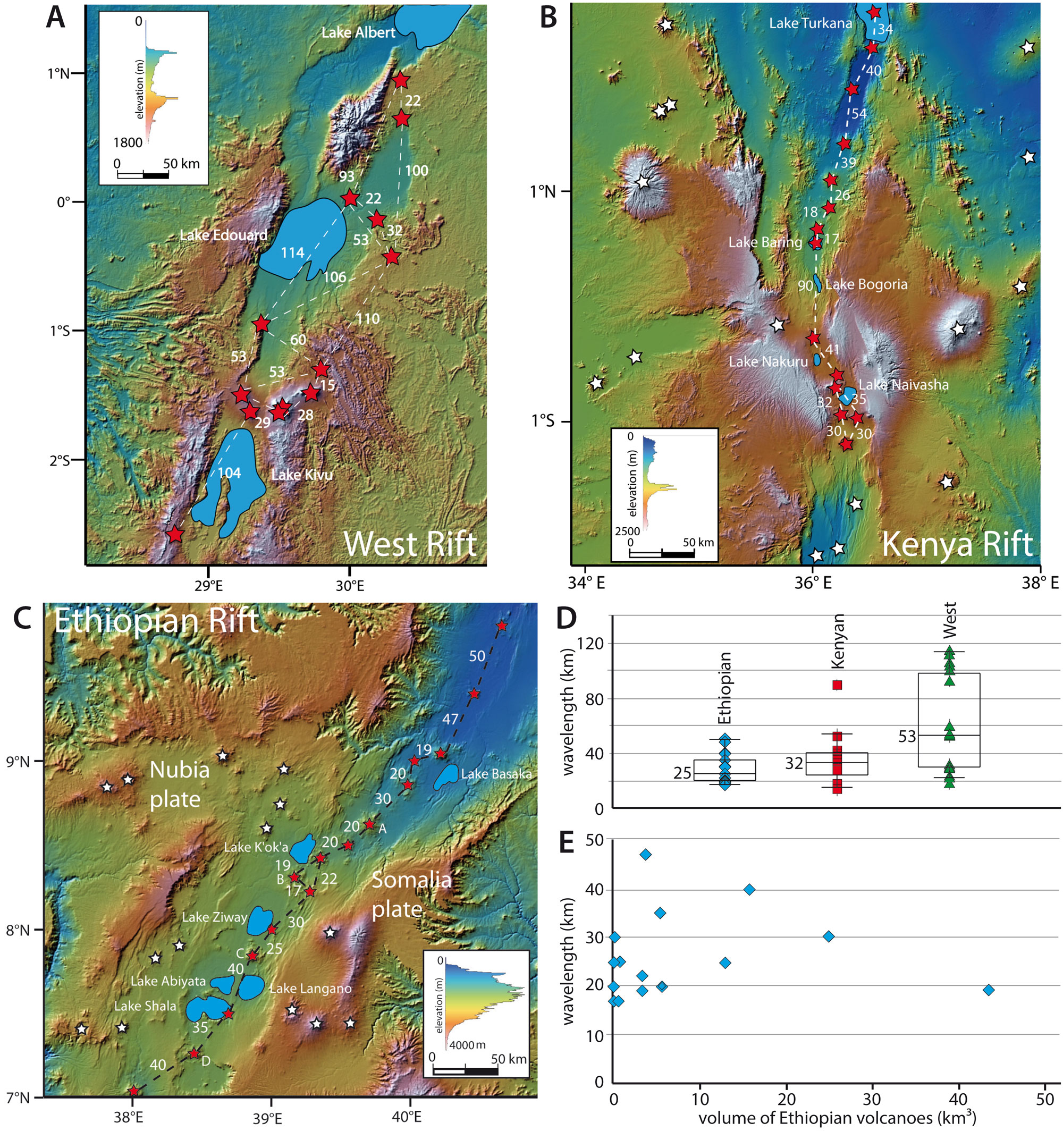
Figure 2. The active volcanoes during the last 10 ka of the (A) West, (B) Kenya, and (C) Ethiopian Rift axis. The red and white stars indicate volcanoes centered or offset from the rift axis, respectively. The white number indicates the spacing between volcanoes centered along the rift axis. (D) The median (marked) and inter-quartile range (boxes) in measured volcano spacing increases from the Ethiopian to West sections of the EARS. (E) No consistent relationship exists between spacing and the volume of each volcanoes of the Ethiopian Rift.
For example, spacing of volcanic centers in the MER decreases with increasing volume of the largest volcanoes. However, for smaller volcanoes this trend does not hold; the spacing between volcanoes with a volume ~<10 km3 shows no correlation with volcano volume. Thus, initial volcano formation is likely controlled by deeper processes.
The combination of regular volcano spacing in the Ethiopian and Kenyan Rifts and the presence of relatively warm plume mantle indicate that a Rayleigh-Taylor (RT) instability in the mantle could regulate magma supply along the rift axis. A RT instability occurs in the unstable situation where a dense fluid rests atop a less dense fluid and the interface between them is perturbed; this results in growth of an instability that forms regularly spaced upwelling and downwelling diapirs. The diapirs form at a dominant, or preferred wavelength (i.e., spacing) that is controlled by the fluid parameters (e.g., density contrast, viscosity contrast, layer thickness). For example, when both fluids are Newtonian a larger thickness of the lower fluid layer yields a larger preferred instability wavelength.
To test the hypothesis of a RT instability in the sub-EARS mantle, we developed numerical models of a less dense viscous material (e.g., warm plume mantle) underlying a relatively dense viscous fluid (e.g. non-plume mantle). Simulations are performed with the finite-difference, marker-in-cell code SiStER (Simple Stokes with Exotic Rheologies; e.g., Olive et al., 2016). We simulate the evolution of two fluid layers with different contrasts in density, temperature, and flow law exponent (Newtonian versus Non-Newtonian fluids). We initially perturb the layer interface by 1% of the imposed wavelength and set the box width to half of the imposed wavelength (Fig. 3). By examining a range of parameters, we will be able to address how variations in mantle properties along the Ethiopian and Kenyan rift and between East and West Rifts may control volcano spacing.
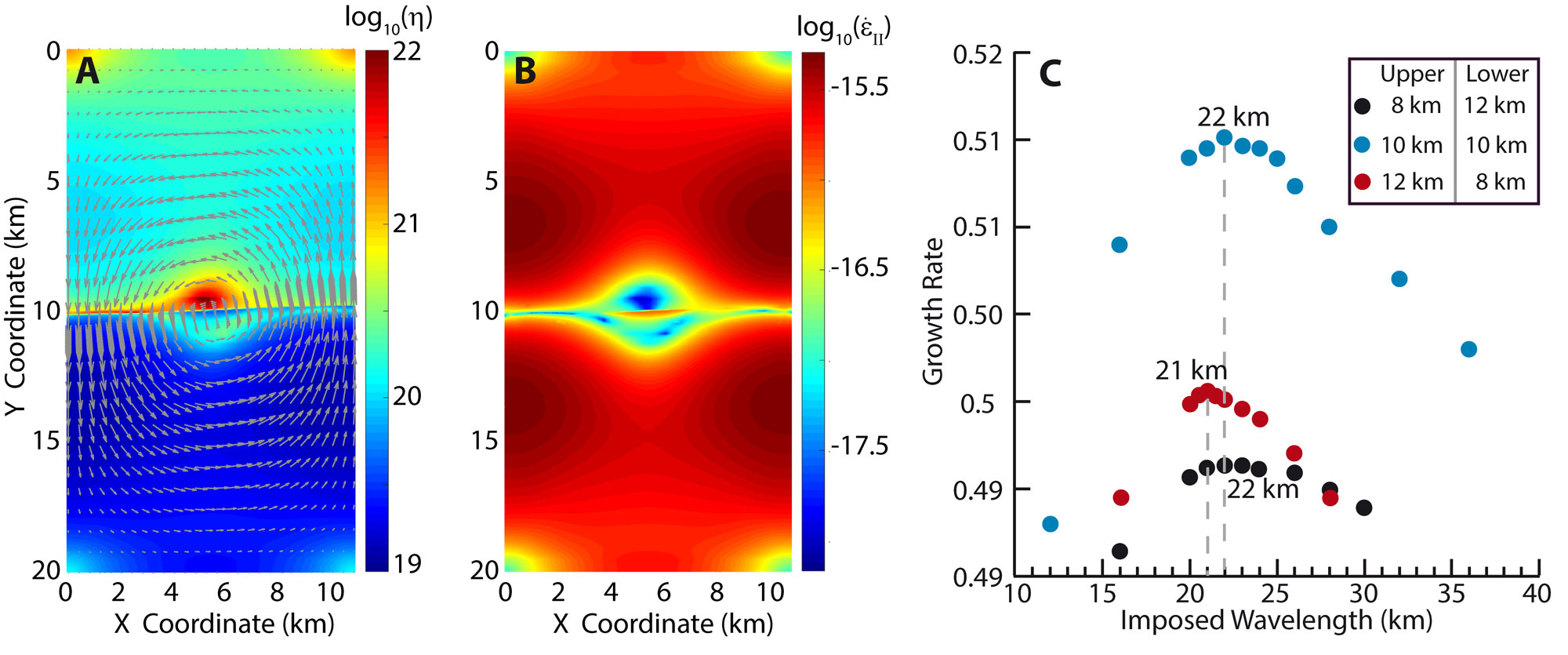
Figure 3. For numerical simulations of non-Newtonian fluids, the (A) viscosity is a strong function of the (B) second invariant of the strain rate field. In contrast to Newtonian cases, these sharp changes in viscosity yield a weak dependence on the (C) thickness of the lower layer (colors) for cases with intermediate layer thicknesses. Gray arrows in (A) are velocity vectors.
Our preliminary results with Non-Newtonian fluids demonstrate that the growth rate of instabilities is not controlled by the lower layer thickness as in Newtonian fluids, but by the characteristic distance over which viscosity changes away from the interface between the two fluids, in agreement with previous studies (Molnar et al., 1998; Miller and Behn, 2012). If the lower layer is significantly thicker than this characteristic distance, than the preferred wavelength of upwelling diapirs will not “feel” the effect of the layer limits. However, if the layer is smaller than the characteristic distance, layer thickness will alter the preferred wavelength. Thus, for relatively thick lower layers, the preferred wavelength depends upon other system parameters, such as the flow law exponent.
For values of the lower layer thickness (~10 km), flow law exponent (3-4), activation energy (E ~200-500 kJ.mol-1), and density anomaly (3000 kg.m-3 in the lower layer and 3200 kg.m-3 in the upper layer) that resemble possible mantle conditions beneath the EARS, we find wavelengths on the order of those for the Ethiopian Rift and portions of the Kenya Rift. Although we have not yet incorporated the effect of background strain rate due to rift extension, spatially variable temperature, and more complicated rheologies (e.g., incorporation of a viscous yield stress), our preliminary results suggest that a RT instability in the upper mantle could conceivably control the volcano spacing along the EARS rift segments. In addition to incorporating the above complexities into our simulations, we plan to compare our predictions to seismic, petrographic, and structural studies in the EARS to further constrain the properties that may be required to form RT instabilities in the sub-rift mantle. ■
Reference informationGeoPRISMS Newsletter, Issue No. 37, Fall 2016. Retrieved from http://geoprisms.nineplanetsllc.com