Kathy Davenport (Virginia Tech) and members of the SSIP field crew
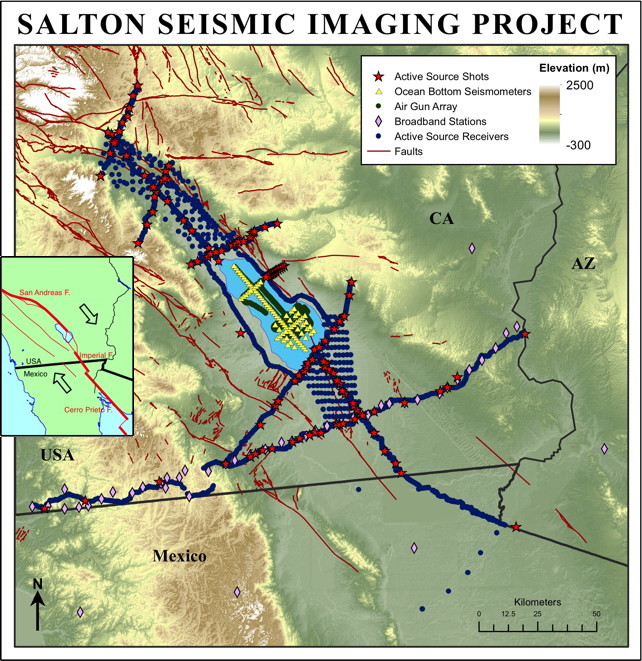
Figure 1. SSIP Project map. Red lines are faults; symbols (see index) are seismic sources or seismographs.
In early 2011, the Salton Seismic Imaging Project (SSIP) descended on Southern California. The Salton Trough was part of the Gulf of California focus area for MARGINS, and processes in this setting also address issues of rift initiation and evolution (RIE) important to GeoPRISMS. Over the course of three weeks, we acquired refraction and low-fold reflection seismic data along 7 lines totaling over 750 km, two 3D grids, and an offshore array. About 130 people participated in the data acquisition, including students from 31 different colleges and universities. During this time, 126 shots were fired, totaling 33,329 kg of explosives, and a 3.4-liter GI airgun was fired 2330 times in the Salton Sea. These sources were recorded on land on 2595 single-component seismographs and186 three-component seismographs at 4235 unique sites, as well as 48 three-component ocean bottom seismographs at 78 sites in the Salton Sea. A 42 station broadband deployment was also live during this time. We deployed instruments in sand dunes and snow, on bombing ranges and golf courses, beneath windmills and Joshua trees. We hiked through mesquite, avoided cactus and endangered lizards, and endured the stench of the Salton Sea. It took the best efforts of all the people involved to accomplish this massive data acquisition in the Salton Trough!
The Salton Trough is a prime target for investigating rift initiation and evolution and earthquake hazards because it is the northernmost extent of the Gulf of California extensional province. The San Andreas Fault ends in southern California, and strike-slip plate motion is transferred to the Imperial Fault. This step-over created the Salton Trough, a basin extending from Palm Springs to the Gulf of California. Previous studies suggest that North American lithosphere has rifted completely in the central Salton Trough. However, rifting here has been strongly affected by rapid sedimentation from the Colorado River, preventing the onset of seafloor spreading as has occurred in the southern Gulf of California. The 20-25 km thick crust in the central Salton Trough apparently is composed entirely of new crust created by magmatism from below and sedimentation from above. Between the major transform faults, active rifting is manifested by faults observed in modern sediment, abundant seismicity, minor volcanism, very high heat flow, and corresponding geothermal energy production.
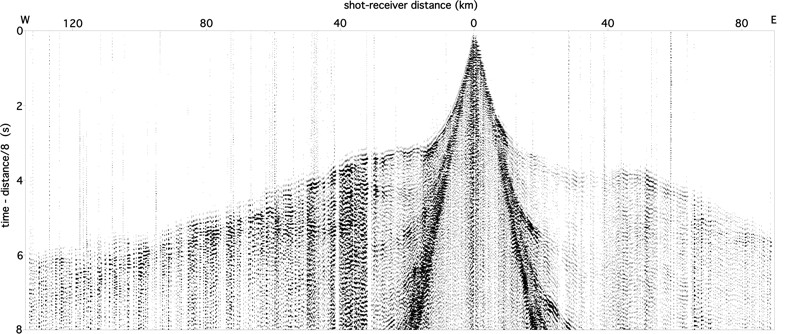
Figure 2. Shot gather. The 911 kg shot was at the Imperial Fault. The 1142 seismograms (from Texans, plus vertical components from RT130’s) were recorded along Line 2 that extends from the San Diego and Tijuana suburbs across the Peninsular Ranges, Salton Trough and Chocolate Mountains, to the Colorado River.
Based on the paleoseismic record, the southern San Andreas Fault is considered overdue for an earthquake of magnitude >7.5, and other nearby faults have had historic earthquakes with magnitudes >7. Earthquake hazard models and strong ground motion simulations require knowledge of the dip of the faults and the geometry and wavespeed of the adjacent sedimentary basins, but these parameters are currently poorly constrained.
SSIP ultimately will constrain the initiation and evolution of nearly complete continental rifting, including the emplacement of magmatism, effects of sedimentation upon extension and magmatism, and partitioning of strain during continental breakup. To improve earthquake hazard models, we will image the geometry of the San Andreas, Imperial and other faults, the structure of sedimentary basins in the Salton Trough, and the three-dimensional seismic wavespeed of the crust and uppermost mantle.
Constraining all these targets in the Salton Trough requires good instrument coverage in areas that are not always easily accessible. For instance, the deserts of Southern California are home to multiple military training facilities. These include the El Centro Naval Air Facility, whose bombing ranges are the winter training grounds for the Blue Angels, and the Chocolate Mountain Gunnery Range, Marine lands used for live munitions training. The Navy and Marine Corp were very accommodating to our project, providing safety training and time windows where we could safely cross the bombing ranges to deploy and pick up instruments. Of course, we had to work around the daily operations of these facilities, and that was not always easy.
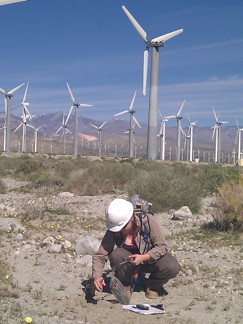
Figure 3. Deploying a Texan seismograph on a wind farm near Palm Springs.
Much of our work in the Imperial and Coachella Valleys was outside the urban areas and farmlands where the population is concentrated. We worked in the desert, the mountains, and on the Sea. Very often we found ourselves driving in washes or hiking because there were no roads where we needed to be. Bushwhacking, boating, and travelling cross-country led to many adventures for our deployment crews.
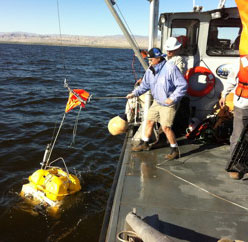
Figure 4. Deploying an OBS into the shallow Salton Sea.
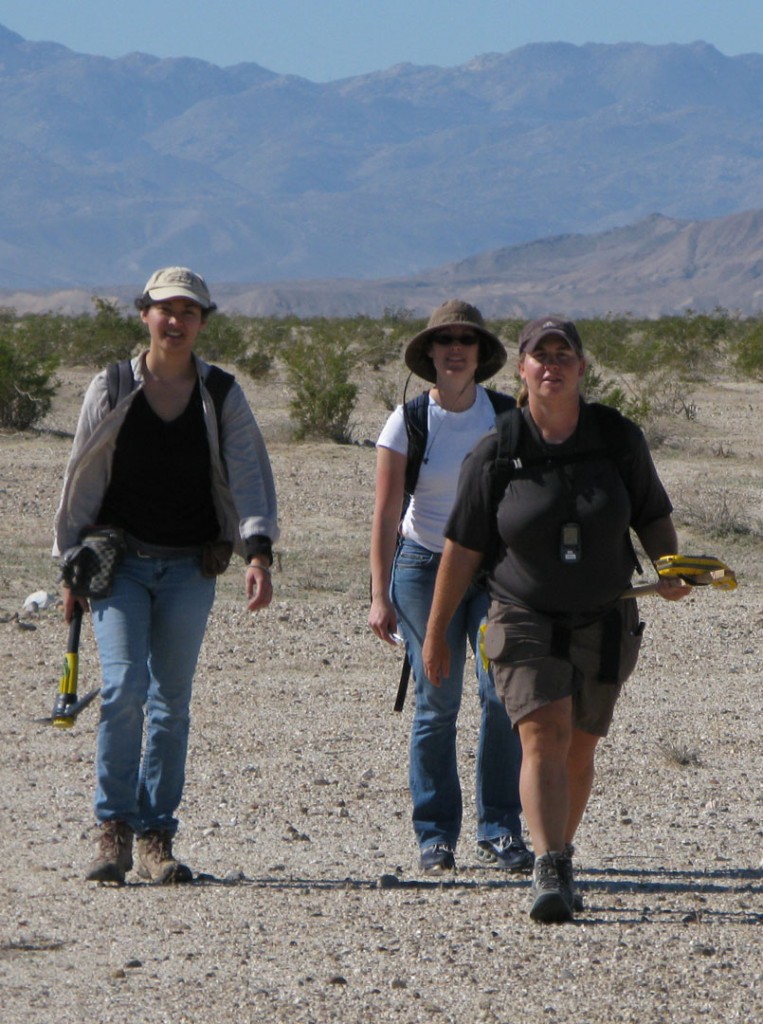
Figure 5. Backpacking seismographs across a Naval bombing range. Each person is carrying about 8 Texan seismographs and deployment equipment.
Onshore SSIP principal investigators are John Hole (Virginia Tech), Joann Stock (Caltech), and Gary Fuis (USGS, Menlo Park), working with Mexican collaborators Antonio Gonzalez-Fernandez (CICESE) and Octavio Lazaro-Mancilla (Univ. Autonoma de Baja California). The onshore work was funded by the NSF MARGINS Program (GeoPRISMS predecessor), the NSF EarthScope Program, and the USGS MultiHazards Program. The marine component, Wet-SSIP, is funded by an NSF Marine Geology and Geophysics Program grant to Neal Driscoll and Alistair Harding (Scripps Inst. Oceanography) and Graham Kent (Univ. Nevada, Reno). Broadband-SSIP is led by Simon Klemperer (Stanford Univ.) with funding from the NSF Geophysics Program. Onshore seismometers were provided by the EarthScope FlexArray and IRIS PASSCAL instrument pools with field support from PASSCAL. The OBSs were supplied by the OBSIP.
“Report from the Field” was designed to inform the community of real-time, exciting GeoPRISMS -related research. Through this report, the authors expose the excitement, trials, and opportunities to conduct fieldwork, as well as the challenges they may have experienced by deploying research activities in unique geological settings. If you would like to contribute to this series and share your experience on the field, please contact the GeoPRISMS Office at info@geoprisms.nineplanetsllc.com. This opportunity is open to anyone engaged in GeoPRISMS research, from senior researchers to undergraduate students.
We hope to hear from you!
GeoPRISMS Newsletter, Issue No. 28, Spring 2012. Retrieved from http://geoprisms.nineplanetsllc.com