Anne Bécel
Lamont-Doherty Earth Observatory, Columbia University
During September-October 2014, the NSF-GeoPRISMS-funded Eastern North American Margin (ENAM) Community Seismic Experiment (CSE) collected deep penetration multichannel seismic (MCS) reflection profiles covering a 500 km wide section of the Mid-Atlantic passive margin offshore North Carolina, which formed after the Mesozoic breakup of supercontinent Pangea The ENAM-CSE data extend farther offshore than previous seismic surveys conducted in this area and encompass the full transition from continental breakup to mature seafloor spreading while specifically providing unique constraints on the events surrounding the final stage of continental rifting and the initial stage of seafloor spreading, which remain poorly understood. The results shown here demonstrate the ability of MCS data to image four distinct domains that highlight different basement characteristics and provide new insights on the degree of extensional strain localization experienced during continental breakup and how the earliest oceanic crust was formed after rifting.
Introduction
The Eastern North American Margin (ENAM) is a passive continental margin that was formed by the rifting of the Pangaea supercontinent and the opening of the Atlantic Ocean during the Late Triassic and Early Jurassic.
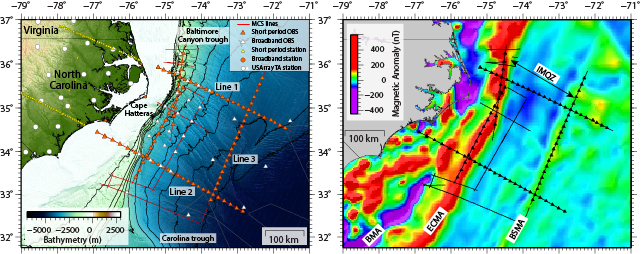
Figure 1. a) Elevation Map (Andrews et al., 2016) contoured every 500 m showing the location of the ENAM Community Seismic Experiment. Line 1 and ENAM Line 2 were chosen to characterize the deep structure of the Carolina Trough south of Cape Hatteras, and the Baltimore Canyon Trough north of Cape Hatteras, respectively whereas the Line 3 was chosen to characterize the structure of the crust and uppermost mantle to better understand the origin of the Blake Spur Magnetic anomaly. b) Magnetic anomaly map (Maus et al., 2009) of the North American Margin. ECMA: East Coast Magnetic Anomaly; BSMA: Blake Spur Magnetic Anomaly; IMQZ: Inner Magnetic Quiet Zone.
From offshore Nova Scotia to Florida, the ENAM has been classified as a volcanic-type margin (Marzoli et al. 1999). Multichannel seismic profiles have imaged seaward dipping reflectors (SDRs) that have been attributed to the subaerial eruption and subsequent subsidence of volcanic flows emplaced during the final phase of rifting (Austin et al., 1990). Seismic refraction profiles beneath the volcanic wedges have revealed a thick sequence of high seismic velocity lower crust rocks interpreted as igneous/magmatic underplating (Holbrook et al., 1994). The East Coast magnetic anomaly (ECMA) is a high-amplitude positive magnetic anomaly running along the length of the margin (Fig. 1) (Keller et al., 1954). The source of the ECMA has been primarily attributed to seaward dipping reflectors in the upper crust (Austin et al., 1990) and is interpreted as the limit between the continental crust and the normal oceanic crust. However, the exact nature and the width of the zone between the continental crust and normal oceanic crust remain uncertain. This zone is thought to either represent a new anomalously thick magmatic crust with higher velocity than lower oceanic crust with no continental crust present (Talwani et al., 1995) or a zone with volcanics on top of magmatic material intruded into extended continental crust or underplated beneath. The nature and the width of this zone are of fundamental importance to understanding the late stage rifting processes and over what time period the continental breakup occurred at this volcanic margin. Margins that experience a voluminous magmatism during rifting tend to have a more rapid continental breakup with a smaller zone of crustal extension (i.e. strain localization) and tend to develop more symmetric conjugate margins.
The Blake Spur magnetic anomaly (BSMA) is a positive, linear magnetic anomaly located 150-250 km to the east of the ECMA (Fig. 1). The BSMA is of lower amplitude than the ECMA but also consists of segments with several magnetic peaks separated by troughs. The age of BSMA is unknown but extrapolated ages range between 168-173 Ma. The nature and origin of this magnetic anomaly is still debated and different models have been proposed. BSMA is either thought to mark a ridge jump (Vogt, 1973), magmatic pulse associated to a plate re-organization (Klitgord and Schouten, 1986; Kneller et al., 2012) or a change in spreading rate/direction and asymmetry of incipient seafloor spreading during the early opening of the Central Atlantic (Labails et al., 2010). In the ridge jump scenario, the BSMA is thought to represent a sliver of West African rifted continental crust that experienced continental breakup magmatism and that was left on the Eastern North American margin after the early spreading center jumped east of the BSMA. This model implies that a now extinct mid-ocean ridge lies between ECMA and BSMA.
The Inner Magnetic (Jurassic) Quiet zone (IMQZ) lies between the ECMA and the BSMA (Bird et al., 2007). Because the magnetic anomalies are of very low amplitudes and variable in shape, the correlation of magnetic anomalies with magnetic reversals remains challenging in this zone (Fig. 1). Timing and location of breakup at the ENAM thus remain uncertain and the spreading rate of the earliest normal oceanic crust in the IMJQ is not well constrained.
Data acquisition and project goals
This project aims to extract information on the late-stage continental rifting including the relationship between the timing of rifting and the occurrence of offshore magmatism and early seafloor history of the Central Atlantic using multichannel (MCS) data from the ENAM-CSE. The MCS data were acquired on R/V Marcus Langseth using a 6600 cu. in. tuned airgun array and 636 channel 8-km-long streamer. The source and the streamer were both towed at a depth of 9 m for deep imaging. This project involves the multichannel seismic processing and interpretation of two offshore margin normal profiles (450-km-long and 370-km-long, respectively), spanning from continental crust ~50 km off the coast to mature oceanic crust 110 km east of the BSMA and a ~350-km-long MCS profile along the BSMA (Fig. 1). These primary MCS lines are also coincident with the ENAM seismic refraction profiles recorded on ocean bottom seismometers.
Results
The high-resolution MCS data provide detailed structure of the sedimentary cover and crust (Fig. 2 and Fig. 3). The initial images of the two margin normal profiles reveal several major changes in the basement character and roughness between the ECMA and the BSMA (Fig. 2) that have not been previously described. The four domains described below correspond to distinct magnetic anomalies that suggest that magnetization contrasts exist between those domains. The interpretation of the new observations from MCS data give new important insights into the late stage of rifting and rift to drift transition.
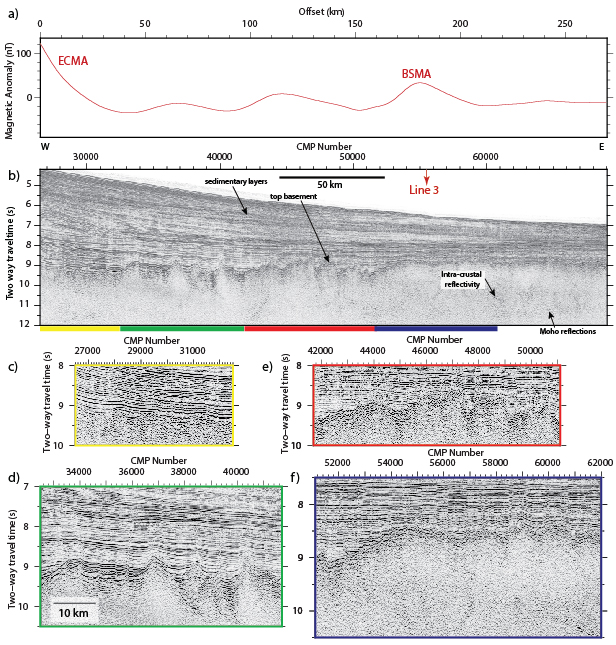
Figure 2. a) Magnetic anomaly profile coincident to the seaward part of ENAM-MCS Line 2 (Maus et al., 2009). b) Post-stack time migrated profile of the seaward part of ENAM-MCS Line 2 c) d) e) f) zooms into the four different domains discussed in the text and that display different basement characteristics.
From CDP 26500 to CDP 32500 (Fig. 2a and 2c), the top of the basement is smooth and less reflective than on the seaward part of the profile and it is also less distinguishable from the sedimentary layers above. The top basement characteristics suggest that it could correspond to smooth volcanic flows emplaced in shallow water conditions and coincide with the landward onset of the ECMA.
From CDP 32500 to CDP 41700 (Fig. 2a and 2d), there is a step up in the basement and a drastic change in the basement roughness. In this area, the crust is highly tectonized by normal faulting forming tilted, faulted crustal blocks. This crust could be interpreted as highly extended continental crust due to the geometry of syn-rift sedimentary sequences in the basement half-grabens. This interpretation would be in conflict with the zone between the continent and the oceanic being purely magmatic and would suggest that continental crust could have been thinned by faulting before being intruded by igneous material. Alternatively, this crust could be oceanic crust formed at very slow spreading rates (<15 mm/yr). Very slow-spreading crust is known to be fragmented by normal faulting with large crustal blocks (long wavelengths). On the sole basis of basement architecture, we cannot fully support either of the two proposed hypotheses. Ocean-bottom seismometer (OBS) refraction data acquired during the ENAM-CSE and coincident with the MCS data used in this project will help to decipher the nature of the crust where tilted basement blocks are imaged.
From CDP 41700 to CDP 51100 (Fig. 2a and 2e), the basement roughness appears to be that of a typical oceanic crust formed at a steady state slow spreading ridge.
From CDP 51100 to CDP 62000 (Fig. 2a and 2e), starting at the BSMA anomaly and seaward, the top basement is very smooth and reflective and the BSMA anomaly appears to coincide with a step-up in top basement.
Along the BSMA, clear Moho (Mohorovic Discontinuity) reflections are observed 2.5-3 s (8.12-9.75 km assuming an average crustal velocity of 6.5 km/s) beneath the top basement (Fig. 3) and are relatively continuous. Abundant intracrustal reflections, primarily restricted within the oceanic lower crust, are also observed over crust formed at BSMA time but also in younger crust.
In the ridge jump scenario, the BSMA would represent thinned continental crust intruded by igneous material. However, the top basement is very reflective indicating a strong impedance contrast between the sediment layers and the top basement. This would be more in agreement with a top basement produced by submarine seafloor spreading at a mid-ocean ridge than subaerial or shallow water emplacement of volcanics within sediments that would reduce the impedance contrast as in Fig. 2c.
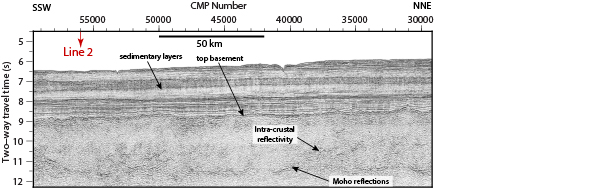
Figure 3. Part of pre-stack time migrated profile (ENAM-MCS Line 3) along the Blake Spur Magnetic Anomaly.
The layering imaged within the lower crust (Fig. 2f) could indicate magmatic intrusives but the well-developed Moho would suggest no underplating. In addition, lower crustal reflections persist in younger crust beyond the BSMA suggesting that this crust is not continental crust that experienced pervasive melt migration during extension. There is also no evidence of a fossil spreading center between ECMA and BSMA.
A drastic increase in seafloor spreading rate and a change in the spreading in the vicinity of the BSMA could explain the change of the basement smoothness from rough to smooth and the basement relief but would not explain the thicker than normal oceanic crust. A magmatic pulse at BSMA time would produce a strongly magnetized upper oceanic crust and could explain the magnetic anomaly. The magnetic pulse would also be in agreement with the thicker than normal oceanic crust and smooth basement topography observed in the data.
The outcomes of the project described above clearly show that the MCS data from the ENAM-CSE provide important information for the study of late-stage rifting processes at this margin. Ultimately, results will be integrated with the landward part of the profiles (not shown here).
This project involves collaboration with Brandon Shuck and Harm van Avendonk at UTIG who are working on the offshore wide-angle reflection/refraction modeling coincident to the multichannel seismic lines used in this project. By combining constraints from the multichannel seismic profiles, refraction modeling and potential field studies, we hope to better understand implications for variations in crustal structures, faulting and magmatism seen in the MCS data at this margin and at a broader scale expand our knowledge of the continental breakup and early seafloor spreading at passive margins worldwide. Results from this project will also be integrated with two others GeoPRISMS projects recently awarded that aim to examine other datasets from the ENAM-CSE. ■
Reference information